Theacrine Study
Theacrine is popular in the sports nutrition circles among bodybuilding athletes and fans. Many of our clients ask us to offer more information on study and research. However, there is no much useful news or documents for theacrine. Here is one good evidence:
The central nervous system activities of theacrine (1,3,7,9-tetramethyluric acid), a purine alkaloid which is abundantly present in Camellia assamica var. kucha, were investigated in ambulatory activity, pentobarbital-induced sleep and forced swimming test in mice, compared with two other purine alkaloids, caffeine and theobromine. Caffeine treatment led to a marked increase in the ambulatory activity accompanied with decreasing of the immobility time in forced swimming test at both 10 and 30 mg/kg. Under the same conditions, neither theacrine nor theobromine showed obvious excited efficacy. Both doses of theacrine could significantly prolong the sleeping time induced by pentobarbital, while caffeine and theobromine e xhibited an inverted effect. These results indicated that theacrine possessed potent sedative and hypnotic properties and its central nervous system effects were different from those of caffeine and theobromine.
1. Introduction of theacrine
We have long known that tea is the most popular beverage in the world. All types of tea including green tea, oolong tea and black tea are manufactured from the same plant species, Camellia sinensis L., which was first discovered in China where it has been used as a daily beverage, and has been known to have beneficial effects on health for thousands of years.
The most familiar purine alkaloids, caffeine and theobromine, are present in large quantities in camellia sinensis. Although theacrine (1,3,7,9-tetramethyluric acid) (figure 1) was first discovered in 1937 as a minor purine alkaloid in camellia sinensis leaves, little attention was paid to it until the end of the last century. Zhang et al. found that some of the endemic tea plants, Camellia assamica var. kucha, grown in wild woodland at 1370 m altitude in Yun-nan province of China, contained larger quantities of theacrine. It is well known that caffeine is the most widely consumed behavioural active agent in the world; it affects human psychomotor and cognitive performance including increase of alertness, energy and ability to concentrate. Theobromine, as the immediate precursor of caffeine in tea leaves, also has psychoactive properties, but its effects may be milder than those of caffeine even though it has a similar chemical structure. Previous research suggested that adenosine receptor blockage was responsible for purine alkaloids’ diverse CNS effects, and the differences in effects resulted from different affinities with adenosine receptors. Theacrine is an important purine alkaloid converted from caffeine by hydration, oxidation and methylation, though its influence on CNS has not been widely studied. Therefore, we investigated the behavioural effects of theacrine by means of ambulatory activity, pentobarbital-induced sleep and a forced swimming test in mice.
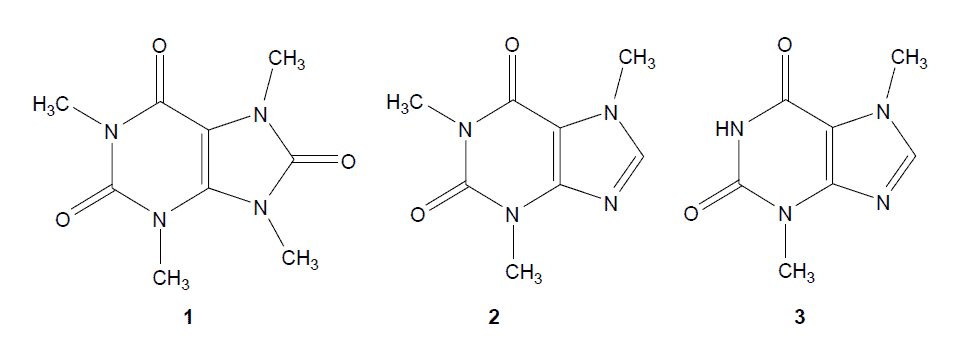
chemical structure of theacirne, caffeine,theobromine
Figure1. Chemical structures of theacrine (1,3,7,9-tetramethyluric acid, 1),
caffeine (1,3,7-trimethyxanthine,2) and theobromine (3,7-dimethylxanthine, 3).
2. Results and discussion
2.1 Analysis of purine alkaloids in camellia assamica var. kucha by reverse-phase high-performance liquid chromatography (RP-HPLC)
The quantification of caffeine and theobromine was made using standard calibration curves for marketed compounds. Theacrine was quantified using a calibration curve derived from theacrine isolated from C. assamica var. kucha. Complete baseline separation of alkaloids was achieved by an isocratic HPLC procedure in 20 min, as illustrated in figure 2A and B. The concentrations of purine alkaloids in two kinds of tea are shown in table 1.
2.2 Effects of theacrine on ambulatory activity
Figure 3 shows that 10 mg/kg of caffeine increased the mouse’s ambulatory activity within 1 h test duration, while theacrine and theobromine were able to produce a remarkable reduction in the activity. 30 mg/kg of caffeine caused a marked increase in ambulatory activity in the whole test procedure and reached its maximum level at 30 min after administration. The same dose of theacrine and theobromine treatment did not lead to any significant effect on the ambulatory activities of mice.
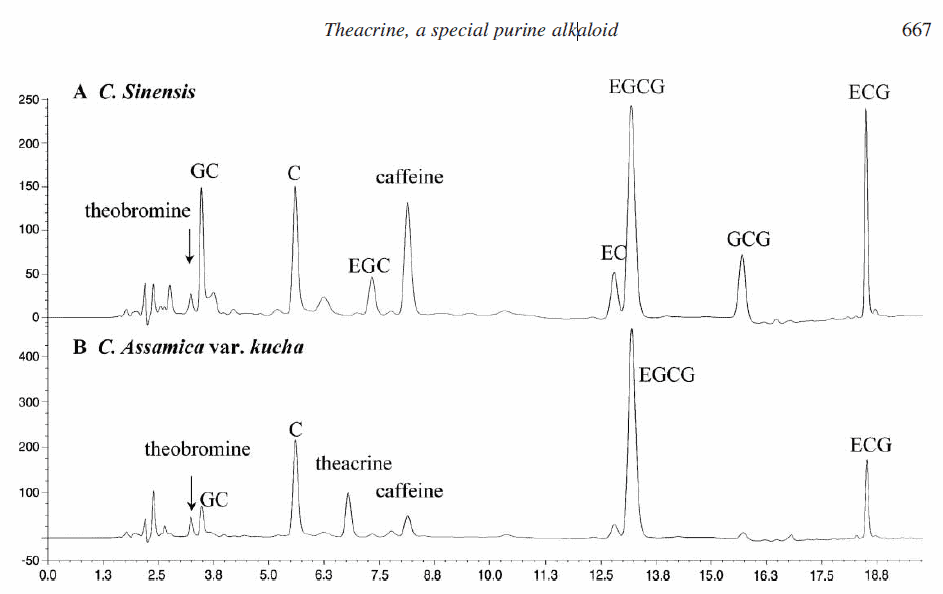
major purine alkaloids and polyphenols in Cammelia assamica var. kucha
Figure 2. The major purine alkaloids and polyphenols present in C. assamica var. kucha leaves (A) and C. sinensis leaves (B). C, (þ)-catechin; GC, (2)-gallocatechin; EGC, (2)-epigallocatechin; EC, (2)-epicatechin; EGCG, (2)-epigallocatechin gallate; GCG, (2)-gallocatechin gallate; ECG, (2)-epicatechin gallate.
Table 1. Quantitative analysis of theacrine, caffeine and theobromine in C. assamica var. kucha.
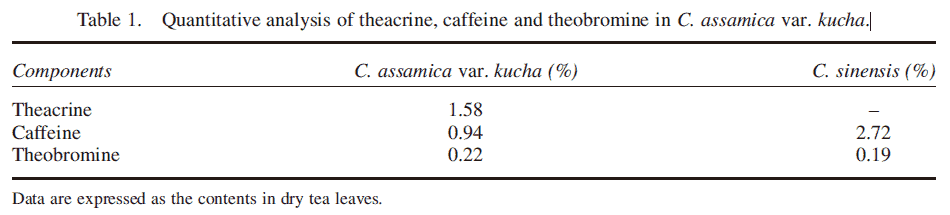
Quantitative analysis of theacrine, caffeine and theobromine in C. assamica var. kucha
2.3 Effects of theacrine on pentobarbitone-induced sleep time
As depicted in figure 4, a comparison with control mice fed with water, sleep time was significantly decreased in the group of caffeine-fed mice and the result revealed that caffeine reliably increased their arousal level. Theobromine also reduced pentobarbital-induced sleep time in mice slightly, while eliciting no significant change. Theacrine, on the other hand, prolonged remarkably pentobarbital-induced sleeping time at both doses.
2.4 Effects of theacrine on behavioural despair test by forced swimming
Figure 5 is a representation of the rotatory locomotor activity of samples in the forced swimming test. Caffeine showed a clear dose-related diminution of the immobility time, while both doses of theobromine increased the duration of immobility in mice slightly. Neither dose of theacrine had notable effects on the rotatory locomotor activity in the same procedure. The results demonstrated that caffeine reliably possessed a significant stimulant effect, while the central nervous activities of theobromine were much poorer and even reversed compared with caffeine. Although theacrine did not exhibit an obvious stimulant effect in both ambulatory activity and forced swimming test, it remarkably prolonged the pentobarbitalinduced sleeping time of mice. Yacoubi and Holtzman reported that the central stimulatory effect of purine alkaloids could be related to blockage of adenosine receptors [15,16].
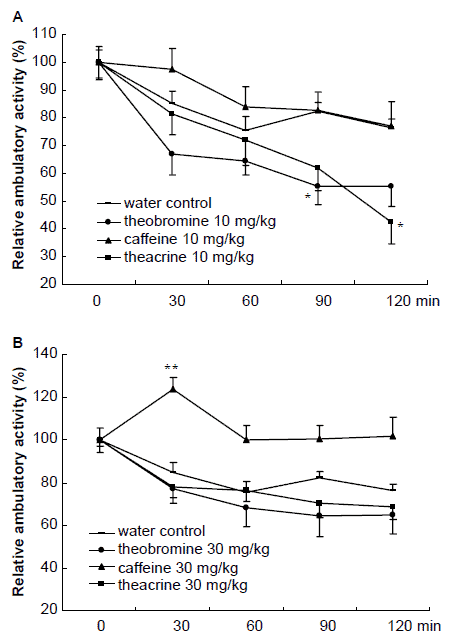
Effects of theacrine, caffeine and theobromine
Figure 3. Effects of theacrine, caffeine and theobromine on the ambulatory activity in mice at 10 mg/kg (A) and 30 mg/kg (B). Activity was monitored for 10 min, and at an interval of 30 min, for a total of 120 min. The substances were administered at t=0. Results are expressed as ±S.E.M. (n=24 or 25). Each point represents the relative ambulatory activity to its corresponding activity at t=0. *P<0.05, **P<0.01 vs. water control group at each time interval.
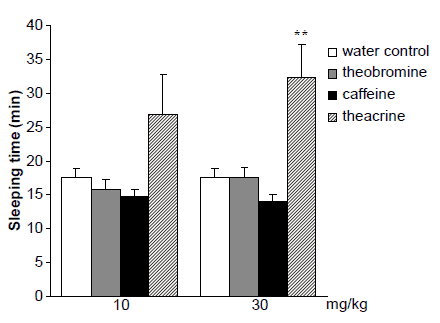
Effects of theacrine, caffeine and theobromine on sleeping time
Figure 4. Effects of theacrine, caffeine and theobromine on sleeping time induced by pentobarbital sodium in mice (60 mg/kg i.v.). Results are expressed as means ^ S.E.M. (n ¼ 15). significantly different from the water control group at *P , 0.05 and **P , 0.01.
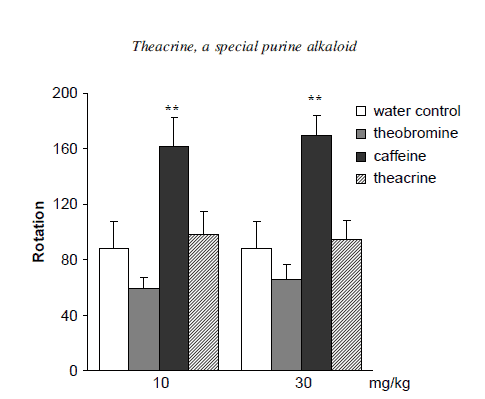
Effects of theacrine, caffeine and theobromine on rotatory locomotor activities
Figure 5. Effects of theacrine, caffeine and theobromine on rotatory locomotor activities during forced swimming test. Results are expressed as means ±S.E.M. (n=20). Significantly different from the water control group at *P< 0.05 and **P<0.01.
Adenosine is likely to be one of the factors that act as endogenous sleep promoters and adenosine A1 and A2A receptors are probably involved in producing the sleep-promoting effects of adenosine [17]. Thus, we have enough reasons to believe that the blockade of adenosine receptors is crucial for the arousal and stimulant effect of caffeine in the present behavioural tests. However, a somewhat novel finding in our present study was that theacrine, as a special purine alkaloid in C. assamica var. kucha, possessed potent central nervous activities different from those of caffeine and theobromine. Since there are differences in purine alkaloids’ interactive effects with adenosine receptors [18], and other neurons such as GABAA [19], monoamine [20] and acetylcholine neurotransmitters [21] are all involved in arousal and ambulation procedures of animals [22], it is difficult to clarify the mechanisms involved in the prolongation of sleep time of theacrine, which still require further study.
3. Experimental
3.1 Plant materials and preparation of theacrine
Tea samples used in this study were collected in May 2005 from wild-grown trees of Cammelia assamica var. kucha at Jin-pin county of Yun-nan province, China. Fresh tea leaves were steamed for 3min, and then put into oven at 808C to dry. 100 g of dry leaves was accurately weighted and extracted twice with 2000 ml of boiling water for 30 min each time. After filtration, the filtrate was concentrated by evaporation under vacuum to 500 ml, then partitioned with 500 ml chloroform three times to afford 1.74 g chloroform extract. The extract was subjected to column chromatography on octadecylsilanised (ODS) silica gel and then eluted with H2O, MeOH/H2O (1:9) and MeOH, respectively. The fraction eluted with MeOH/H2O (1:9) was further separated by preparative HPLC to yield 890 mg of theacrine, 320 mg of caffeine and 21 mg of theobromine.
3.2 Animals and chemicals
Male Kunming mice (18–22 g, Grade II, Certificate No SCXK (Yue) 2003-0002, Med & Lab Animal Centre, Guangdong, China) were housed in groups within plastic cages, kept in a specific-pathogen-free animal room at 23 ^ 18C with a artificial 12:12 h light/dark cycle (lights on from 08:00 to 20:00 h). Food and water were freely available and the animals were acclimatised for 1 week before the experiment. Behavioural studies were carried out between 09:00 and 17:00 H, in a soundproof, air-regulated experimental room where the mice were kept for at least 30 min before each experiment. All procedures involving animals were performed in accordance with the guidelines of the National Institutes of Health on the care and use of laboratory animals. Caffeine and theobromine were purchased from Nacalai Tesque Inc. (Kyoto, Japan), and pentobarbital sodium from Shanghai Chemical Reagent Company (Shanghai, China).
3.3 Analysis of purine alkaloids in C. assamica var. kucha by RP-HPLC
The aqueous extract of C. assamica var. kucha as well as C. sinensis were centrifuged for 15 min at 12,000 xg, the supernatant was filtered through a 0.45-mm nylon-66 filter and analyzed by HPLC with UV detection at 231 nm [23]. The analysis was performed with a Develosil ODS-HG-5 column (4.6×150 mm; Nakalai Tesuque, Kyoto, Japan) at 40°C with a flow rate of 1 ml/min. The mobile phase consisted of two solvent (Solvent A = 95:5:0.05 v/v/v H2O:CH3CN:85% H3PO4; solvent B= 50:50:0.05 v/v/v H2O:CH3CN:85% H3PO4). All solvents used were HPLC grade except for H3PO4 which was analytical grade. The gradient elution program was as follows: 0 min, 10% B; 5 min, 10% B; 8 min, 30% B;10 min, 30% B; 15 min, 80% B; 20 min, 80% B. Purine alkaloids and polyphenols in tea were effectively separated under these HPLC conditions.
3.4 Behavioural evaluation using ambulatory activity in mice
Seven groups of mice were treated with either the substances (10 and 30 mg/kg p.o.) or the vehicle. They were singly placed in a model XZ-4 ambulometer (Chinese Acad. of Med. Sci., Inst. for Material Med., China) Plexiglas cages with four external on–off microswitches placed close to each cage and connected to a multicounter immediately after treatment. The number of horizontal movements was automatically recorded for 10 min, and at an interval of 30 min, for a total of 120 min. Results of the treated groups were compared with those of the water control at each time interval.
3.5 Pentobarbital-induced sleep time in mice
A single dose of pentobarbital (60 mg/kg) was injected i.v. to mice 30 min after the oral treatment with the examined substances or the vehicle. The sleeping time, defined as the time elapsed between the disappearance and recovery of the righting reflex, was measured with a chronometer and expressed in minutes.
3.6 Evaluation of behavioural despair by forced swimming test
When mice were exposed to a situation of forced swimming in a restricted space, they made an active attempt to escape, after a period of vigorous activity and gradually then become passive and immobile. The method of forced swim test developed by Porsolt et al. was based on the observation of the total duration of immobility which was considered when the mice made no further attempts to escape except the movements necessary to keep their head above the water during the whole test session. The method we used was similar to the previous reports which have documented that climbing movements using forepaws were more related to attempt to escape than swimming. So there is a possible correlation between the rotatory locomotor activities of mice during swimming as assessed by the number of climbing tours, and an antidepressant-like or excited effect. The test device (OHC O’Hara & Co. Ltd, Japan) include five polycarbonate cages (length: 25 cm; width: 7 cm; height: 24 cm) and a multi-counter. The cages were filled with water up to the middle of the rotatory wheel (18 cm), and the water temperature was maintained between 22 and 23°C. In a pre-test session, the mice were placed into the cages individually and forced to swim for 10 min, then allowed to return to their cages on 2 consecutive days. On experimental day, the mice were randomly grouped and treated with the three substances (10 and 30 mg/kg, p.o.) or the vehicle. A total of 20 min after the treatment, each animal was placed into a cage again for 6 min and the total rotation of wheel was recorded during the last 5 min of a single 6-min test session. The mean result obtained with each group was compared with the control group.
3.7 Statistical evaluation
The data were expressed as mean±S.E.M. Statistical differences were calculated by one-way ANOVA, followed by Dunnett’s post hoc analyses comparisons between individual treatment groups and controls. In all cases, P<0.05 was taken as the level of significance.
References
[1] C.D. Wu, G.X. Wei. Nutrition, 18, 443 (2002).
[2] Q.Y. Zhu, R.M. Hackman, J.L. Ensunsa, R.R. Holt, C.L. Keen. J. Agric. Food Chem., 50, 6929 (2002).
[3] A.G. Dulloo, C. Duret, D. Rohrer, L. Girardier, N. Mensi, M. Fathi, P. Chantre, J. Vandermander. Am. J. Clin.
Nutr., 70, 1040 (1999).
[4] T.B. Johnson. J. Am. Chem. Soc., 59, 1261 (1937).
[5] C.X. Ye, Y.C. Lin, J.Y. Su, X.H. Song, H.D. Zhang. Acta Scientiarum Naturalium Universitatis Sunyatseni,
38, 82 (1999)
[6] B.B. Fredholm, K. Battig, J. Holmen, A. Nehlig, E. Edwin, E.E. Zvartau. Pharmacol. Rev., 51, 83 (1999). [7] N.L. Benowitz. Annu. Rev. Med., 41, 277 (1990).
[8] A. Smith, C. Brice, J. Nash, N. Rich, D.J. Nutt. J. Psychopharmacol., 17, 283 (2003).
[9] R.A. Robson. Am. J. Med., 92(4A), 22S (1992).
[10] D. Shi, J.W. Daly. Cell. Mol. Neurobiol., 19, 719 (1999).
[11] P. Svenningsson, G.G. Nomikos, B.B. Fredholm. J. Neurosci., 19, 4011 (1999).
[12] J.W. Daly, P. Butts-Lamb, W. Padgett. Cell. Mol. Neurobiol., 3, 69 (1983).
[13] V. Ralevic, G. Burnstock. Pharmacol. Rev., 50, 413 (1998).
[14] X.Q. Zheng, C.X. Ye, M. Kato, A. Crozier, H. Ashihara. Phytochemistry, 60, 129 (2002).
[15] M. EI Yacoubi, C. Ledent, J.F. Menard, M. Parmentier, J. Costentin, J.M. Vaugeois. Br. J. Pharmacol.,
129, 1465 (2000).
[16] S.G. Holtzman, S. Mante, K.P. Minnerman. J. Pharmacol. Exp. Ther., 256, 62 (1991).
[17] S. Satoh, H. Matsumura, N. Koike, Y. Tokunaga, T. Maeda, O. Hayaishi. Eur. J. Neurosci., 11, 1587 (1999). [18] P. Svenningsson, G.G. Nomikos, B.B. Fredholm. J. Neurosci., 19, 4011 (1999).
[19] A.K. Mehta, M.K. Ticku. Brain Res. Rev., 29, 196 (1999).
[20] M.G. Hadfield, C. Milio. Life Sci., 45, 2637 (1989).
[21] A.J. Carter, W.T. O’Connor, M.J. Carter, U. Ungerstedt. J. Pharmacol. Exp. Ther., 273, 637 (1995). [22] K.C. Berridge, I.L. Venier, T.E. Robinson. Behav. Neurosci., 103, 36 (1989).
[23] B.J. Xie, H. Shi, Q.Y. Chen, C.T. Ho. Proc. Natl. Sci. Counc. Repub. China (B): Life Sci., 17, 77 (1993). [24] S. Amos, E. Kolawole, P. Akah, C. Wambebe, K. Gamaniel. Phytomedicine, 8, 356 (2001).
[25] R.D. Porsolt, G. Antin, N. Blavet, M. Jafre. Eur. J. Pharm., 47, 379 (1978).
[26] J.A. Siuciak, D.R. Lewis, S.J. Wiegand, R.M. Lindsay. Pharmacol. Biochem. Behav., 56, 131 (1997).